Most of this research comes from Associate Professor Karl Linden and his research group at Duke University in North Carolina. I have made an effort to cut out some of the technical jargon without over-simplifying the findings. I hope you will find it readable and worthwhile. For those who are interested in the detail of the studies, I have included a number of references at the end. These are generally not freely available on the internet, but if you ask me politely (okay…even if you choose to abuse me), I’ll try to get you a copy of the papers that you are interested in. You’ll find my email address by clicking on my profile, above. Okay, here goes…
Ultraviolet (UV) radiation is an effective disinfectant in water and is used widely for this purpose in many developed countries including Australia. The disinfecting properties of UV radiation are due to the ability of certain wavelengths to penetrate the cell walls of microorganisms and be absorbed by the nucleic acids (components on DNA and RNA). The effect may be to either cause the death of the cell or to prevent it from replicating. The portion of the UV radiation band that is most effective for inactivating microorganisms is between about 220 and 320 nm. This is known at the ‘germicidal band’.
UV disinfection is generally more effective than chlorine for inactivation of most viruses, spores and cysts. However effective inactivation some of these organisms require higher UV doses than is used for some disinfection systems aimed primarily at bacterial organisms.
UV lamps for water treatment come in two main types known as ‘Low Pressure’ and ‘Medium Pressure’. Low pressure UV lamps generate radiation at mainly a single wavelength (254 nm), which is close to the most effective germicidal range. Medium-pressure lamps generate radiation at numerous wavelengths within the germicidal band. Only about 7 to 15 per cent of the output is near 254 nm, however the total germicidal output can typically be 50 to 100 times that of low pressure lamps.
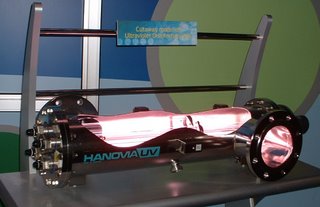
In addition to microbial disinfection, UV radiation can also be used (directly) to break down organic chemicals in water [1; 2]. Organic chemicals are affected by UV radiation at various wavelengths in the 200-300 nm range, with degradation generally dependant on the presence of aromatic rings or double bonds or triple bonds.
In 2000, The Orange County Water District in California detected small concentrations (nanogram per litre) of the carcinogenic chemical nitrosodimethylamine (NDMA) in their Water Factory 21 scheme. The problem was quickly resolved by the addition of a UV treatment process, which rapidly destroys NDMA.
However, for the purpose of breaking down chemicals, UV radiation is more commonly used to promote the initial formation of hydroxyl radicals (HO.). Hydroxyl radicals are about the most powerful oxidising agents known and are much more effective at breaking down chemicals than direct UV radiation. Processes that promote the formation of hydroxyl radicals are known as Advanced Oxidation Processes (AOPs).
UV-AOPs can be achieved by a number of methods including ‘photocatalysis’ with titanium dioxide (TiO2). However the most common method is by reaction of hydrogen peroxide (H2O2) [3-5] by the following reaction:
Oxidation of organic chemicals by hydroxyl radicals is very non-specific and all organic chemicals are ultimately susceptible if sufficient dose is applied [6]. Because of this considerably broader oxidation potential, AOPs can be used to degrade trace amounts of recalcitrant organic chemicals in highly treated effluents.
Once generated, hydroxyl radicals can attack organic molecules by a number of mechanisms. Under suitable conditions, the reaction of hydroxyl radicals with organic compounds may proceed to complete oxidation to produce final products of water, carbon dioxide and salts. This process is known as mineralisation.
The overall extent of oxidation for any AOP is dependant on the contact time and the concentration of ‘scavengers’ in the water (ie non-target oxidisable species). Typically, dissolved organic carbon (DOC) and carbonate/bicarbonate are the most important scavengers in drinking waters or recycled waters. However, pre-treatment processes such as granular activated carbon (GAC) or reverse osmosis significantly reduce DOC concentrations, thus enhancing oxidation efficiency.
In 2004, Linden’s research group published a paper investigating the degradation of some endocrine disrupting chemicals (bisphenol A, ethinyl estradiol, and estradiol) by direct UV photolysis and UV-AOPs [3]. This study included both low pressure UV lamps and medium pressure UV lamps. It revealed that without enhanced hydroxyl radical formation, medium pressure lamps are required for the degradation of these contaminants. However, regardless of which type of lamp was used, the EDCs were much more effectively degraded using UV/H2O2 advanced oxidation. The chemical degradation rate constants for the AOP process were on the order of 10,000,000,000 /M/sec. This means that given suitable initial water quality (achieved by pre-treatment) and sufficient UV/H2O2 dose, these AOP processes can be an extremely effective barrier for these chemicals in water recycling schemes. Some results from the study are shown below (click for a larger image).

[1] Rosenfeldt, E. J., Melcher, B. and Linden, K. G. (2005) UV and UV/H2O2 treatment of methylisoborneol (MIB) and geosmin in water. Journal of Water Supply Research and Technology-Aqua, 54(7), 423-434.
[2] Shemer, H., Sharpless, C. M. and Linden, K. G. (2005) Photodegradation of 3,5,6-trichloro-2-pyridinol in aqueous solution. Water Air Soil Poll., 168(1-4), 145-155.
[3] Rosenfeldt, E. J. and Linden, K. G. (2004) Degradation of endocrine disrupting chemicals bisphenol A, ethinyl estradiol, and estradiol during UV photolysis and advanced oxidation processes. Environ. Sci. Technol., 38(20), 5476-5483.
[4] Shemer, H. and Linden, K. G. (2006) Degradation and by-product formation of diazinon in water during UV and UV/H2O2 treatment. J. Haz. Mat., 136(3), 553-559.
[5] Shemer, H., Kunukcu, Y. K. and Linden, K. G. (2006) Degradation of the pharmaceutical Metronidazole via UV, Fenton and photo-Fenton processes. Chemosphere, 63(2), 269-276.
[6] Shemer, H., Sharpless, C. M., Elovitz, M. S. and Linden, K. G. (2006) Relative rate constants of contaminant candidate list pesticides with hydroxyl radicals. Environ. Sci. Technol., 40(14), 4460-4466.
2 comments:
Thanks Stuart. I would be interested to read posts like this for all of the various “multiple barriers” that have been proposed for schemes like Toowoomba and Goulburn. Keep it up!
thanks a million for these precious informations
Post a Comment